Blog
Getting real: Your EV’s real-world range and emerging best practices
During winter, whenever I drove between my home in the San Francisco Bay Area and Lake Tahoe for a ski trip, I noticed the range of my battery electric vehicle was less than it typically is in the city. That was the result of a few different factors, including driving in low ambient temperatures at higher speeds, and similar kinds of real-world differences in fuel efficiency are common with traditional internal combustion engine vehicles. So, it’s not just electric vehicles (EVs). But as EVs are beginning to enter the mainstream, it’s important to understand real-world range, and a new ICCT report sheds light on this and highlights opportunities for regulators and drivers.
The study was systematic and based on real-world data from the open lab of China’s National Big Data Alliance of New Energy Vehicles (NDANEV). We chose to analyze the 10 best-selling battery electric passenger car models in China from 2017 to the end of 2021. Then we looked at their anonymized operations data from full calendar year 2021 in five cities with different climates, Shenyang, Beijing, Hangzhou, Chengdu, and Guangzhou. This yielded over 140,000 vehicles in total. (Because data privacy restrictions prevented us from obtaining the raw data, the open lab of NDANEV processed the raw data based on the methodology we specified and shared aggregated data. We did not independently verify the data because we have no access to the raw data.)
The difference between real-world and nominal range, which is the range from the type-approval certification that is shared with consumers, in five kinds of real-world conditions is shown in Figure 1. We defined daily average ambient temperature of ≤ -7 ℃ as “very cold,” ≤ 0 ℃ as “cold” (this is all temperatures that are ≤ 0 ℃, including ≤ -7 ℃), 30–35 ℃ (> 30℃ and ≤ 35 ℃) as “hot,” and > 90 km/hr as “high-speed” driving conditions. “All conditions” is the median value of all trips and conditions in our sample, without controlling for temperature, speed, city location and typology, or other factors. The car models are anonymized, but their class is shown in parentheses.
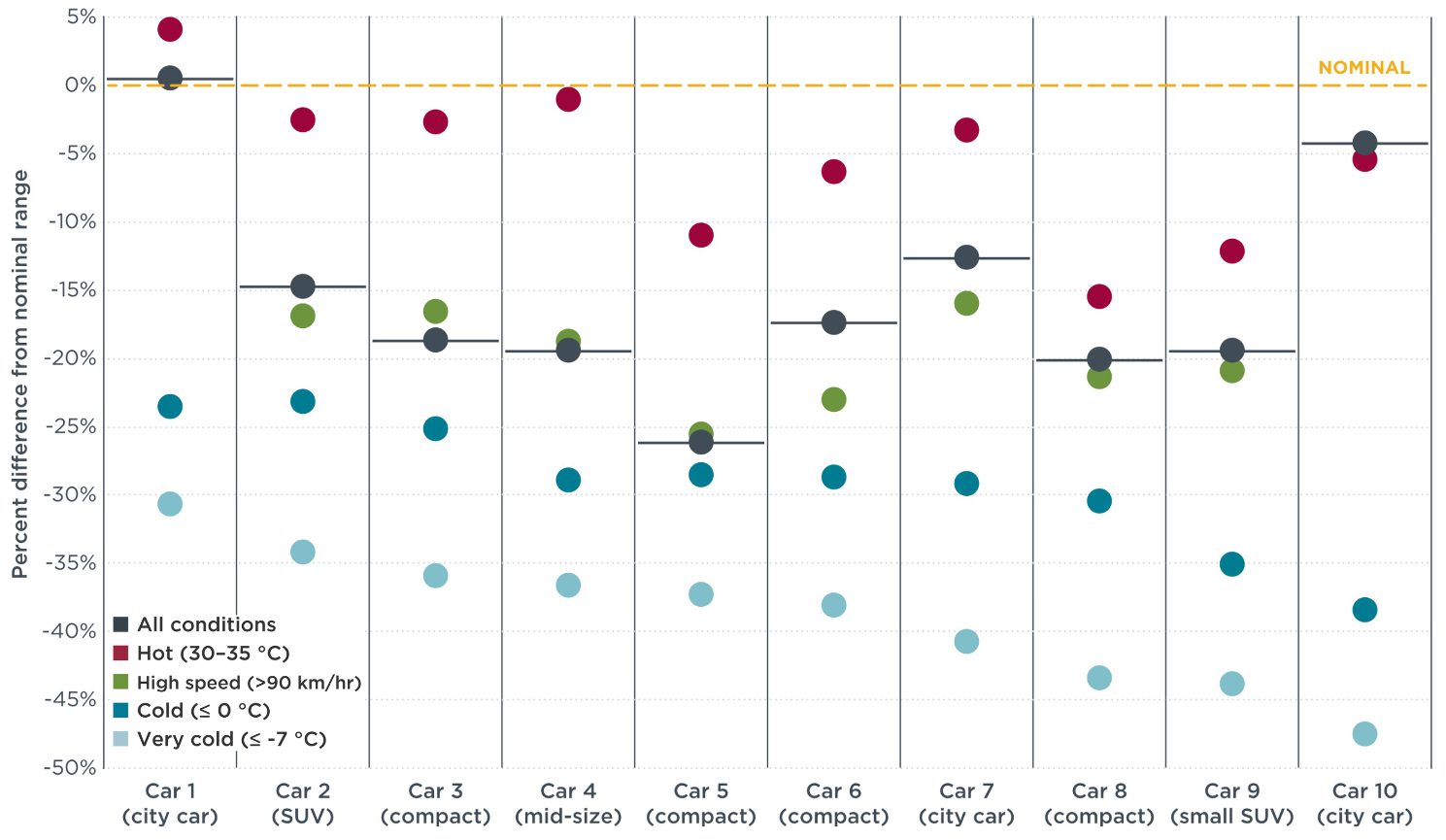
Figure 1. Difference between real-world and nominal range by model, with vehicle class shown underneath. Models are listed left to right based on performance in “very cold” conditions and there are missing data points for high-speed conditions due to limited data under such conditions.
Except for Car 1, ranges under “all conditions” were below the nominal ranges, meaning that the vehicles were sometimes unable to travel as far on a single charge as nominal values suggest. On average, range under “all conditions” was 15% lower than nominal. Multiple factors could have contributed to Car 1’s better alignment with nominal range, including generally better performance under high and low ambient temperatures; infrequent high-speed driving, likely because it’s a city car that’s mostly used for daily commute; and fewer vehicles deployed in colder cities like Shenyang and Beijing.
Other highlights from our analysis include:
- Low ambient temperature had the most adverse impact on range. The reduction in range varied from 30%–50% in “very cold” conditions and from 20%–40% in “cold” conditions.
- The reduced range from high-speed driving was between 15% and 25%.
- High ambient temperature both increased and decreased average range, and its impact was less significant than low ambient temperature or high-speed driving. High temperature increased range by 5% for one model but generally reduced it, likely due to the use of air conditioning; the largest reduction was 15%.
To reduce the gap between nominal and real-world range and to better inform drivers of such gaps, we offer some recommendations to governments, manufacturers and dealers, and consumers.
Government: Incorporate test cycles that account for real-world conditions such as low temperatures (≤ -7 °C), higher speeds, and the use of air conditioning, so that the nominal values better reflect on-road performance. Incorporating energy consumption and range values under the various real-world driving conditions in efficiency labels for EVs could also help to bridge the current information gap. As part of such labeling programs, governments could regulate the types of information used for manufacturer advertisements (see next paragraph).
Manufacturers and dealers: While continuing to improve EV technology to extend range, manufacturers and car dealers could proactively improve methods to estimate real-world performance and then provide consumers with their best estimates, both at the sales stage and during use (i.e., reflect any improvements on the remaining range estimate on the dashboard). They could also provide clear instructions and recommendations for optimal battery storage and operation.
Consumers: Just like internal combustion engine vehicles, proper use and maintenance of EVs contributes to better performance, and this is especially true of good charging and storage habits, which prolong battery life. For example, it is generally recommended to avoid overcharging or over discharging your car beyond the 20%–80% state of charge zone, storing your car in extreme temperatures (e.g., above 60 ℃ or below -30 ℃, based on some companies’ manuals), and storing your car in a low state of charge for a prolonged period.
Our study for passenger cars was published alongside a sister study for heavy-duty vehicles in China. These are the first where ICCT analyzed such a rich data set of EVs and their operations data. Using this kind of real-world data to guide proactive actions like the ones mentioned above would better inform consumers about real-world EV range, whether it be during a winter ski trip or a summer road trip in hot weather. This could boost consumer confidence and reduce uncertainty about performance. Both of these could support faster and wider adoption of EVs, which is vital for achieving goals of reducing air pollution and greenhouse gas emissions.